Architects don’t build without modeling. They create “blueprints,” produce renderings, and build 3D models. But while these planning tools may resemble the actual building in shape, there is no resemblance in size or materials. As a result, except in the case of manufactured or modular buildings, the finished product will be the first time that real building materials have come together in exactly that configuration. That is one of the reason that architecture tends to be conservative in its rate of change. Without real-world testing, big change is risky.
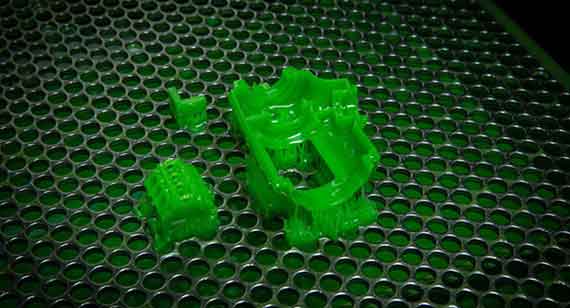
In many cases, their value proposition is their innovation, the fact that they are different from anything that has existed before. And they have to be developed and rolled out quickly to beat competitors to market. Smart prototyping can support all of those goals; the challenge is choosing the right prototyping processes at each point in development.
Product development is different. Today’s products are designed to be manufactured in thousands or hundreds of thousands, and both parts and assembled products can be built and tested throughout the development process. That, in part, explains today’s high rate of product innovation. But it also puts a lot of pressure on the prototyping process. New products have to meet or exceed buyer expectations in a very competitive market.
What is 3D Printing?
3D printing is a process that uses digital CAD models to build physical, often layered, real-life objects. The appropriateness of the technology depends on the application of the part. A concept model of a brain, for example, has inherent medical value to a doctor during surgical planning, but it would never go into production because only one or two printed pieces are needed in a plastic-like material. Other times, additive manufacturing might be used to create fully functional end-use parts in small quantities of engineering-grade metals.
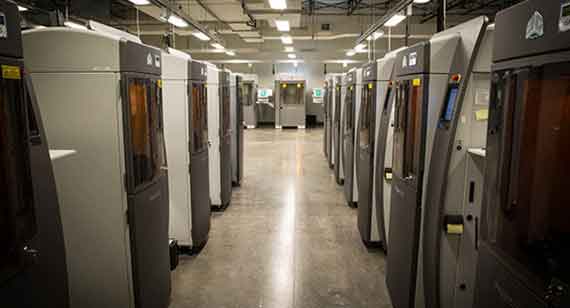
However, if manufacturing will eventually entail a process like injection molding, 3D printing will have more limited use in development. In the later stages of development of a cast or molded part, for example, it will be important to test parts that are identical (or nearly so) to final production parts. This will involve injection molding plastic or metal prototypes in a repeatable fashion. Thus, the method of prototype manufacturing can shift during the development process depending on application, material requirements, manufacturability and other factors.
Early-stage prototypes are typically produced in very small numbers and don’t necessarily have to hold true to all the functional characteristics of production parts. Since material selection and internal structure of the part are not as critical at this stage, prototypes can be produced using a variety of additive technologies that are both fast and affordable.
Uses of parts produced by additive processes include:
- Production parts
- Functional models
- Visual aids
- Fit and assembly testing
- Tooling patterns and components
- Jigs and fixtures
- Concept models
- Patterns for casting
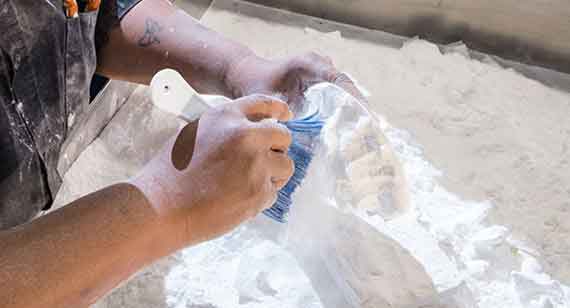
How to Choose an Additive Manufacturing Technology
Different prototyping methods serve different purposes. Take, for example, a designer or engineer who is developing a handheld device containing moving parts. The development process might begin with a series of 3D CAD models. These allow quick creation and virtual assembly of the components. When ready for first physical prototypes, the designer might produce additive prototypes from the CAD models, choosing SL prototypes for the shell components for that method’s superior surface finish and SLS prototypes for the internal components for that method’s good material properties. As development proceeds, there might be several iterations using those processes as the shell and internal part designs evolve.
When it comes time for functional testing—seeing how the internal parts perform under load and how the case withstands being dropped—the designer might initially send out the 3D CAD models to have one or several prototypes of each component machined from appropriate materials. These prototypes would have the physical characteristics of production parts and, particularly in the case of the shell, duplicate the cosmetic appearance. For larger-scale testing, the same CAD models could be used to produce rapid injection-molded parts for physical and market evaluation. If testing indicated that the product was ready for the market, those same molds could be used to produce parts for market while steel tooling was being milled for high-volume production.
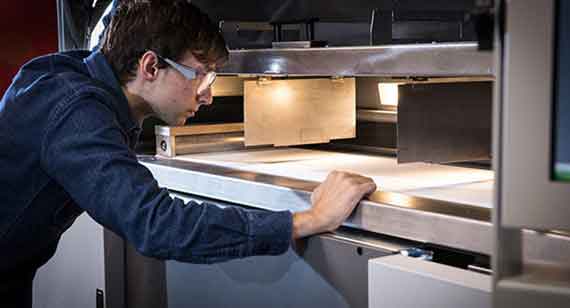
What are the Advantages of Additive Manufacturing
Building a part in thousands of thin layers affords those designing CAD models for 3D printing the opportunity to create highly complex geometries that are often impossible to mold—internal channels and holes that are unreachable by end mills, or entire assemblies printed as a single piece. But what happens when additive prototypes are ready to graduate to injection molding? Moving from stainless steel prototypes built by direct metal laser sintering (DMLS) into low-volume metal injection molding (MIM) works as a good product development example. Whereas the importance of molding-specific design considerations like draft, radii and uniform wall thickness are minimal in 3D printing, once a shift is made into MIM, these elements become much more critical. At China Metal Parts, automated software identifies moldability issues and recommends solutions in an interactive quote. That might mean a bit of design retooling, but it can quickly turn a printed prototype into a production-ready part.
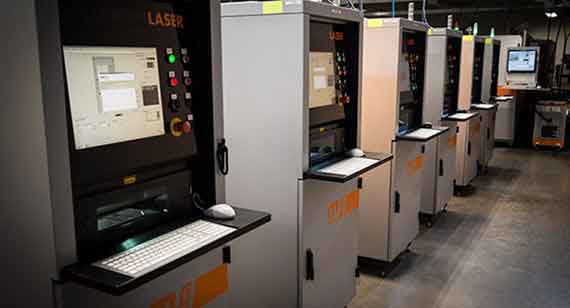
Economies of Scale
Additive processes all share the common impracticality of mass production into the thousands and tens of thousands. Is a new frontier of additive scalability an area that additive could soon explore? According to Terry Wohlers, from independent consulting firm Wohlers Associates, Inc., medical and aerospace industries are beginning to embrace increased printed part production as well as companies in dental and jewelry fields. For a seismic shift to occur, it will likely take more than that. Currently, 3D printing plastic materials are typically 50 to 100 times more expensive than traditional manufacturing, according to Wohlers, not 50 to 100 percent more. So presently, low quantities are suitable for additive manufacturing. When equipment throughput increases and equipment and material decrease, the potential for larger production volumes will increase. Until then, processes like injection molding—that involve an initial tooling investment, but lower per-part price as quantities increase—remain a logical next step after prototyping.
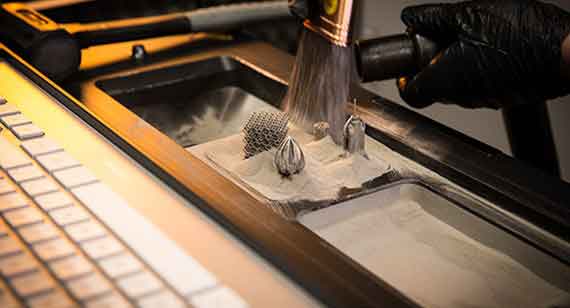
An Overview of Additive Manufacturing Processes
There isn’t necessarily a preferred additive prototyping process. The challenge is finding the best prototyping methods for your project and for each phase of your project. Variables among prototyping methods include speed, cost, appearance, supported materials and a variety of physical characteristics. In some cases, all you need is something you can hold in your hand; in others, fit with other components is required.
BJET |
Binder Jetting |
---|---|
![]() |
Binder jetting is one of the simplest and most basic additive prototyping processes. An inkjet print head moves across a bed of powder, selectively depositing a liquid binding material, and the process is repeated until the complete part has been formed. After completion, the unbound powder is removed leaving the finished object. Pros
|
FDM |
Fused Deposition Modeling |
---|---|
![]() |
Fused deposition modeling (FDM) melts and re-solidifies thermoplastic resin (ABS, polycarbonate or ABS/polycarbonate blend) in layers to form a finished prototype. Because it uses real thermoplastic resins, it is stronger than binder jetting and may be of limited use for functional testing. Pros
Cons
|
SLA |
Stereolithography |
---|---|
![]() |
Stereolithography (SLA) uses a computer controlled laser to build parts in a pool of UV-curable resin. As each layer is drawn by the laser, the part is lowered in the pool of liquid resin allowing the next layer of liquid to be solidified. Quality of the finished part depends largely on the quality of the equipment and process used. Pros
|
SLS |
Selective Laser Sintering |
---|---|
![]() |
Selective laser sintering (SLS) employs a computer controlled CO2 laser to fuse layers of powdered material such as nylon from the bottom up. Strength is better than that of SL but lower than that produced by subtractive processes like injection molding or CNC machining. It also has some use as a production method. Pros
|
PJET |
PolyJet |
---|---|
![]() |
PolyJet (PJET) uses a print head to spray layers of photopolymer resin that are cured, one after another, using ultraviolet light. The layers are very thin allowing quality resolution. The material is supported by gel matrix that is removed after completion of the part. Elastomeric parts are possible with PolyJet. Pros
|
DLP |
Digital Light Processing |
---|---|
![]() |
Digital light processing (DLP)-based additive manufacturing digitally slices a solid into layers, which a Texas Instruments DLP chip projects, one after another, onto the surface of a liquid photopolymer bath. The projected light hardens a layer of liquid polymer resting on a movable build plate. The build plate moves down in small increments as new images are projected onto the liquid, hardening each subsequent layer to produce the finished object. The remaining liquid polymer is then drained from the vat, leaving the solid model. The process can be useful for producing low volumes of small, highly detailed parts but is less suitable for larger parts, especially those requiring smooth finishes. Pros
Cons
|
DMLS |
Direct Metal Laser Sintering |
---|---|
![]() |
Direct metal laser sintering (DMLS) is the leading additive method for making metal prototypes. It is similar to selective laser sintering of plastic resin, but is suitable for use with metals including aluminum, stainless steel, titanium, cobalt chrome and Inconel. It offers good accuracy and detail, and excellent mechanical properties. DMLS can be used for very small parts and features, and because it is an additive process, it can reproduce geometries that might be impossible to machine such as enclosed spaces. Layers can be as thin as 20 microns, and tolerances on small features can be as small as ±0.002 inches. Secondary operations on parts produced by DMLS can include machined drilling, slotting, milling and reaming, and finishing procedures including anodizing, electro-polishing, hand polishing, and powder coating or painting. Pros
|
Outsourced Prototyping
While a few of the processes described can be carried out in-house, the majority of this kind of prototyping is outsourced. Outsourcing allows the developer to choose the best methods for any particular need. That can entail using multiple prototyping methods over the course of a single project. In selecting a vendor, consider the needs and goals of your project:
- Can the manufacturer provide suitable prototyping methods for your specific needs?
- Can it help you select the best method at each stage of the process?
- Does it offer any kind of design assistance?
- If you need a series of prototypes, can the manufacturer provide continuity?
- How experienced is the manufacturer in the processes you will use?
- Can it produce the maximum quality available for each prototyping method?
- If necessary, can it provide secondary operations for your prototypes?
- If material is critical, what materials can the manufacturer offer in the selected method, and if a particular method cannot utilize your preferred material, can it offer other methods?
- What turnaround times does it offer?
- What is the manufacturer’s reputation for meeting deadlines?